Here's a deep dive into the world of motion control amplifiers. Making the right design choice can have a big impact on your machine's cost and performance.
In this article we take a look at how to select, or design, the right amplifier for your unique application.
In the last twenty years, the world of amplifiers has gone through a sea change. Amplifier modules and chips now routinely attain hundreds of Watts of output per cubic inch – even up to a kilowatt or above. This is due to advances in the high-efficiency of power switching electronics as well as the availability of dedicated current controller ICs.
Beyond sheer power, motion amplifiers for positioning and velocity control applications now routinely provide advanced phasing algorithms that get your motor spinning faster, more smoothly, and more efficiently than ever before. How will these advances affect your next design?Read on and find out!
The power and the glory
Motion systems consist of three major components: the controller, the amplifier and the motor/actuator. The controller is an IC (integrated circuit), board, or box that outputs control signals to the amplifier. The format of this control signal varies depending on the motor type. For servo motors, it is generally the desired voltage or current (also often referred to as desired torque) encoded as a +/- 10V analog signal. For step motors the control signals are most often digital pulse & direction signals.
The amplifier accepts these control signals and converts them into higher power motor coil voltages. One, two or three electrical coils are driven by the amplifier depending on the motor type. DC Brush motors have one coil, Step motors have two, and Brushless PM (permanent magnet) motors have three. The amplifier may also provide functions such as short circuit protection, over-temperature protection, current control, and commutation.
Figure 1: Generalized motion control architecture block diagram
Finally, the motor converts current into force and movement. Note that by motor, we include not just rotary and linear motors, but a broader range of actuators such as voice coils.
For the purpose of this article we will focus on precision motion control only. For amplifiers, this means proportional four quadrant control. That is, the ability to command gradated output levels of both acceleration and deceleration in both the negative and positive directions. Proportional four quadrant control is the standard for positioning applications, as well as precision velocity or torque control applications.
Behold The Motion Control Amplifier
What exactly does a modern motion control amplifier do? Most engineers understand that at the core, amplifiers accept low-power control signals and generate higher power voltages to drive the motor.
But there are actually numerous functions provided by motion control amplifiers. Knowing which of these are important for your application represents 90 percent of the battle over what type of motion control amplifier you need. In the next several sections we provide a summary of this. Then, once we know what amplifiers can do, we will dive wholeheartedly into the actual amplifier choices available for your next design.
Amplification
By far and away the dominant approach toward amplification for motion control is a technique known as switching. Switching amplifiers take advantage of the inherent efficiency with which MOSFETs (Metal Oxide Field Effect Transistors) and IGBTs (Insulated Gate Bipolar Transistors) can selectively apply a bus voltage to a circuit load.
In the context of motors this means that when the switch is on, the supplied motor voltage is fed directly to the motor coil.
There are a few schemes for controlling the timing of these on and off periods. PWM (Pulse Width Modulation) is a common approach, where the frequency stays the same but the duty cycle is varied. Figure 2 shows this, illustrating PWM (Pulse Width Modulation) signals at various duty cycles.
Figure 2: PWM (Pulse Width Modulation) timing diagram
Despite the overwhelming prevalence of switching amplifiers for motion control, there are still some applications where non-switching linear amplifiers (sometimes called transconductance amplifiers) are needed. Although 3 to 5 time times less efficient than switchers, linear amplifiers generate much less EMI (Electromagnetic Interference) and thus find use where that characteristic is critically important.
Current Control
Current control, also often called torque control in servo motors, means the active regulation of current flowing through each coil of the motor.
Why is this needed? The short answer is inductance. Motor coils are inductors, which means they inherently resist changes in current. This effect is called self-inductance. To improve response times an active current controller compensates by driving the coil at a higher voltage until the desired current is reached, then lowers the voltage to keep it at that current.
The faster the response time, the higher the current bandwidth, and the faster the system can do useful things like home in on a target position, react to a velocity perturbation, etc. So when it comes to current bandwidth, faster is better.
Beyond self-inductance, the motor itself is a highly dynamic electromagnetic environment that induces back-EMF (Electro Motive Force) onto the coil as it spins. This effect increases as spin increases, as does the tendency of the motor to act as a generator during deceleration. All of these effects can seriously distort the actual current through each coil compared to the voltage that is applied to it.
In many amplifiers current control is accomplished using a PI (Proportional Integral) loop. The basic elements are the desired current, an electronic device to measure the actual current in the coil, and a PI controller which operates on the difference between the desired current and the measured current (the current error). Such a control loop is shown in Figure 3.
Figure 3: PI (Proportional, Integral) current loop flow
Older amplifiers change P and I manually via screws. Modern amplifiers (digital amplifiers) allow these values, and many others, to be set digitally and stored into the amplifier.
There are numerous current control schemes beyond the PI loop. Another broad class of controller regulates the switch's off time. Popular in step motor applications, these amplifiers are called fixed off-time PWM controllers.
So when is current control critical? For step motors, the answer is essentially 'always'. This is because accurate current control in steppers affects nearly every aspect of performance; top speed, positioning accuracy, heat generation, and more.
Step motors also have a very high 'pole count' (the number of electrical cycles per mechanical rotation), which means to get the motor to rotate at a high speed you need a high current bandwidth.
In DC Brush and Brushless PM motor applications, current control may not be needed every time, but it is increasingly rare that it not be used. Current control improves reaction times, acceleration & deceleration rates, top speed, and efficiency. It also provides important safety functions. Happily, as electronics and current sensing hardware has become less expensive, the cost of current control has diminished.
Phasing
Phasing here means the selective application of the voltage or current to the coils in multi-phase motors such as step motors, which most often have two phases but which sometimes have three or five phases, and Brushless PM motors, which nearly always have three phases. Proper phasing keeps these motors moving forward or backward as desired by the host controller.
With step motors, various phasing schemes are given various names, such as full stepping, half stepping, and microstepping. This is shown in Figure 4, which shows various waveforms for a two-phase step motor.
Figure 4: Full step, half step, and microstep waveforms for two-phase step motors
The most basic commutation scheme for Brushless motors is called trapezoidal, or six-step drive. As the motor rotates, special sensors called Hall effect sensors provide continuous information on the rotor angle. There are six unique Hall-encoded states from the three Hall sensors. Each state represents a 60 degree portion of a full 360 degree electrical cycle.
Trapezoidal drives apply a 'positive', 'no drive', or 'negative' command to each motor coil depending on which of the six encoded Hall states the motor rotor is positioned in. Figure 5 shows this, along with waveforms that other commutation schemes provide.
Figure 5: Six-step and sinusoidal Brushless DC commutation waveforms
Trapezoidal commutation generally works well but has discontinuities at the Hall transition points. This increases torque ripple and vibration, and may complicate positioning, particularly if the desired position happens to be near a Hall transition point.
The next major step up in commutation is called Field Oriented Control (FOC), also known as vector control. Figure 6 shows the control flow of this more complicated, math-intensive, scheme.
FOC can be used with Hall sensors, but is more often used with position encoders because they provide hundreds or even thousands of resolvable locations per motor rotation. When this granularity of position is fed into the FOC engine, the resulting coil waveforms are significantly more continuous and smoother than trapezoidal commutation.
Figure 6: Control flow for sinusoidal and FOC (Field Oriented Control) current loops
At the core of FOC are mathematical transforms called Park and Clarke transforms. The Park and Clarke transforms alternately 'de-reference' and 're-reference' the control frame from the A, B, C 'coil frame', to a rotation-invariant frame known as the d (stands for direct), q (stands for quadrature) frame.
By performing this algorithmic tour de force, current control becomes isolated from the rate at which the motor is spinning. This is hugely beneficial to operating at high speed because current control is normally strongly affected by motor rotation.
When are the fancier flavors of phasing needed? For step motors, the step resolution directly correlates to the position resolution, so more tends to be better, up to about 64 microsteps per full step. Beyond that, the motor has trouble reacting even if the electronics are capable of higher resolutions.
For Brushless DC motors, basic six-step trapezoidal commutation may work well for some applications, however, FOC-based control will provide benefits for applications that need smoother motion, faster response times, more efficient motor operation, and higher speed.
But wait, there's more
As it turns out there are a number of additional functions provided by amplifiers. To keep this deep dive to a reasonable length I will just touch upon them here. You can do some further digging on your own, or just give Performance Motion Devices technical support department a call if you have specific questions.
As it turns out, neither Brushless PM nor step motors respond in a perfectly linear manner to sinusoidal waveforms. Sometimes to get the most accurate motion, you may want to modify the control waveforms. This is called waveform shaping, and is most commonly used with step motors to improve final endpoint position accuracy. For Brushless motors, shaping the waveform can linearize the torque output, reducing ripple.
Another area that you should keep your eye on is fault detection. There are many types of amplifier faults that can be detected, and generally, more is better. Look for detection/protection from over temperature faults, short circuit fault, over current fault, bus overvoltage and under voltage fault, and more.
I2t current management is a general technique, related to fault detection, for limiting total current output. But rather than controlling the instantaneous maximum current (which is itself a nice feature available on many amplifiers), I2t allows you to control the total energy delivered in units of amp2sec. This provides a more useful protection mechanism for both the amplifier and the motor because it is current over a period of time that causes heating, not just instantaneous current. Figure 7 illustrates this, showing what happens to output control signals when an I2t overcurrent condition occurs.
Figure 7: I2t current foldback event parameter processing
Earlier in this deep dive we mentioned that when an axis decelerates, the motor may become a net energy generator. Applications such as spindles, centrifuges, pumps, and blowers frequently encounter this condition. When this occurs excess energy may get 'pushed' back onto the bus voltage to the point of damaging other equipment connected to the bus. To handle this, some amplifiers provide power management such as a dedicated output signal that controls a shunt resistor.
Performance Trace in your amplifier may be important for applications where efficiency, motion smoothness, and transfer time is key. This is an area where modern digital amplifiers excel. At their core are digital circuits that can rapidly record information such as commanded current vs. actual current, temperature, bus voltage, and many other parameters. There are many such products, but Performance Motion Devices' Atlas amplifiers are representative, allowing trace of simultaneous parameters at up to 20 kHz. When coupled with Windows-based motion capture software you can display or store the results for analysis. A representative screen capture for such a trace function is shown in Figure 8.
Figure 8: Example motion trace screen capture screen from Pro-Motion Developer Software
Beyond all of these features there are of course the basic specifications associated with the amplifier. These are the voltage range, continuous and maximum current rating, and the environmental conditions such as the temperature operating range. Needless to say, you want to select an amplifier that has enough get up and go for your application!
With this primer behind us, we are ready to start discussing amplifier choices. We will move from low power to high power, although several of these amplifier categories reach broadly across the entire power spectrum.
Chips ahoy!
Figure 9: Dedicated single axis amplifier IC
A large class of machine controllers can utilize dedicated single axis motor amplifier ICs to provide amplification. Such motion control ICs are provided in various bridge configurations such as H-bridges (used with DC Brush and step motors) as well as half-bridges (used with Brushless DC motors).
Basic entries in this amplifier category provide just the switching function and perhaps some overcurrent protection. More sophisticated versions provide digital current control and additional features such as selectable fast decay, slow decay operation.
For step motor control, these ICs directly input pulse and direction signals and provide half step as well as microstepping control. They utilize current control techniques especially suited to the demands of step motor control.
There is also a good measure of choices for driving Brushless PM motors, including 'all in one' ICs for specific end markets like disk drive spindle control and fans. If the motor you plan to use resembles those applications, these ICs may work well for you.
The most important drawback to these ICs is that they rarely top 4 amps in current output or 150W in power output. In terms of drive sophistication, these products are fairly low on the totem pole. If they provide commutation it is typically six-step trapezoidal, and many of the higher level features described above are missing.
Don't have a MOSFET
Figure 10: Intelligent Motor Controller IC - MC73110 from PMD Corp.
The next category of amplifier is designing an amplifier from discrete components. There are a number of reasons you might attempt this, first among them is achieving the lowest possible cost in your application, and raising the power output beyond what single-IC solutions can provide. Other benefits of designing your own amplifier include flexibility, since you can tailor the solution to best suit your application.
With today's powerful semiconductor pre-drivers, switches, or packaged bridges, you will be able to drive 1,000 watts, or multiple kilowatts, in a remarkably small amount of printed circuit board real estate.
The main downside of this approach is that it is relatively complicated, and requires a good knowledge of power switches, current sensing, signal layout, noise management, fault detection, card layout, and other areas. Most of the folks who design their own power amplifiers are compelled to do so by cost considerations, or because their application has some specific need unmet by vendor-provided amplifiers.
Having said that, a nifty new product that has come on the market in the last few years significantly simplifies the task of designing your own motion amplifier. Called IMCs (Intelligent Motor Controllers), these products, provided by several vendors including Performance Motion Devices, bundle complex control tasks such as commutation, field-oriented control (FOC), current sense input, and switch signal generation, into a low cost, compact IC.
Increasing time-to-market pressure and the expanding range of off-the-shelf amplifiers have made the 'roll your own' amplifier choice somewhat less common over the years. But for the right volume, function, and cost point, designing your own amplifier may still make a lot of sense.
I bet you solder that to all the boards
Figure 11: Solderable digital amplifier – Atlas® digital amplifier
The most significant recent development in motion control digital amplifiers is the rise of the solderable amplifier module. Solderable amplifier modules are similar to traditional cable-connected amplifier modules in that a single packaged unit provides all the major functions listed above - amplification, current control, phasing, and many, if not all, of the 'extras'.
They are different in a number of important ways. For starters, particularly for the power, they can output, they are tiny - coming in the 1 to 2 cubic inch range. By way of specific example, Performance Motion Devices' solderable amplifier, called Atlas®, outputs up to a kilowatt of power and is just 1.5" by 1.5" by .6".
Another difference is that by going to a solderable format, interfaces beyond analog +/- 10V become possible. In particular, digital formats are useful because they make it easy to connect with microprocessor-style motion controllers.
Staying digital eliminates the analog conversion step required to generate a +/- 10V torque command, and this same digital interface can be used for setting parameters, pulling outperformance trace data, etc… While there are no standards yet, SPI (Serial Peripheral Interface) is used by some products including Performance Motion Devices' Atlas, RS232 by others.
Finally, eliminating the cables and connectors associated with traditional module-oriented motion amplifiers saves money and improves reliability. Solder joints are far more reliable then cable connections. And because these devices are so small, it is possible to build a single control card that integrates the entire machine controller onto one PC card. That can have a major impact on serviceability and system cost. If the entire machine controller is on a single card, machine servicing consists of swapping out the card.
Solderable amplifiers typically accept voltages in the range of 12 - 60 volts, and offer current outputs up to 15 amps. They are available for Brushless PM motors, DC Brush, and step motors. If you are driving at the higher power output levels you will want to attach the unit to a heat sink or metal frame/cold plate of some kind. Figure 12 shows the approach the Atlas product uses by providing mechanical mounting tabs.
Figure 12: Mechanical drawing of solderable amplifier showing heat sink mounting tabs
The main disadvantage of solderable drives is that (surprise) they must be soldered! This type of solution is appropriate for OEM designs where an application-specific card will be used, but if your volumes don't justify this effort you may be better off with cable-connected amplifiers (discussed in the next section) or motion cards with separate amplifier modules.
Having said that, a good solution to this problem is the use of off-the-shelf motion cards with solderable amplifiers located right on the card. Or alternatively, an off-the-shelf 'piggy back' card with solderable amplifiers that stack on top of the motion card using pre-formatted connectors.
You had me at cable
Figure 13: Cable-connected amplifier module – ION® digital drives
The last major amplifier option we will discuss is the standalone module, which connects by cable to the motion controller. There are two overall flavors; 'dumb' amplifiers which accept a +/- 10V torque signal, and intelligent amplifiers which utilize a digital network connection such as SERCOS, CANopen, Profibus, EtherCAT, PowerLink, or some other standard network interface to connect to the motion controller.
Either way, these modules provide a flexible option for amplification because they can be mounted wherever convenient. Popular choices are in a control rack using DIN rails, near the motor to minimize cable lengths, or even directly on the motor. This last option is called an IMD (Integrated Motor Drive), and has some unique benefits in the area of cable reduction, since encoder signals and Hall sensor signals from the motor feed directly into the IMD and don't have to go back to the controller.
Cable-connected amplifiers modules are provided for all positioning motor types, although for step motors digital network connections are rare, relying instead on pulse & direction signals. They come in a very wide range of voltages and currents, from 10V all the way up to 320V or beyond, and from single digit amps to many dozens of amps.
Feature availability for this amplifier category runs the gamut. Most analog input amplifiers will not have programmable parameters or field oriented control, but they certainly provide fault monitoring and current control.
Moving up the scale, hybrid analog/digital controllers provide an analog +/- 10V command input but utilize a digital core, very often with RS232 connections to program the amplifier parameters. Internally, these amplifiers have digital current loops along with other intelligent programmable features.
Network-based amplifiers tend to be feature-rich, although their functionality is tied to the network protocol. For example, a CANopen amplifier supports the motion command set defined for that network. Vendors often build protocol extensions beyond this standard command set. On the plus side this allows the capabilities of the drive to be extended. On the negative side it makes drives from multiple vendors incompatible with each other.
Summary of selecting the right control amplifier
The key to determining what amplifier choice is best for you is figuring out what features you need for your application. A popular approach here is to buy an off the shelf amplifier with more features than you need, and selectively disable various features to determine the ones that are critical.
Performance Motion Devices, along with a number of vendors, provides excellent Windows-based software that let you accomplish this. As long as the amplifier you are experimenting with provides a trace capability, you can see the results of these changes in glorious detail on the screen. That’s the beauty of digital motion control!
Written by:
Chuck Lewin
President & CEO
Performance Motion Devices, Inc.
You may also be interested in:
- Servo Tuning Deep Dive: Black art, rocket science, or walk in the park?
- Get the most out of your motion controller using Torque FeedForward
- Digital Current Loop Significantly Quiets Step Motor Noise
- Field Oriented Control (FOC) - A Deep Dive
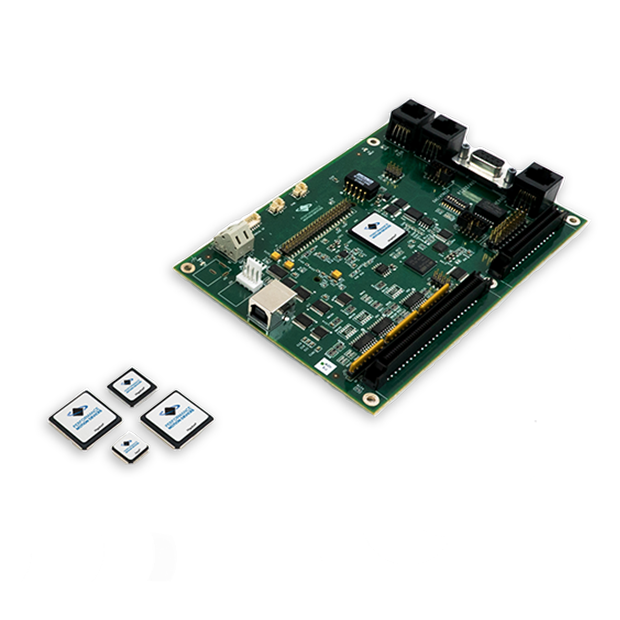
Stay ahead of the competition.
If your existing equipment needs a performance boost or if you need to develop a new product in the shortest development time possible, learn about motion control solutions from Performance Motion Devices.